Ocean is warming up. But why is it so difficult to quantify the amount of heat gained at the ocean surface?
The ocean interacts with the atmosphere via interfacial exchanges of momentum, heat (via radiation and convection), and fresh water (via evaporation and precipitation). These fluxes, or exchanges, constitute the ocean-surface energy and water budgets and define the ocean's role in Earth's climate and its variability on both short and long timescales. However, direct flux measurements are available only at limited locations. Air-sea fluxes are commonly estimated from bulk flux parameterization using flux-related near-surface meteorological variables (winds, sea and air temperatures, and humidity) that are available from buoys, ships, satellite remote sensing, numerical weather prediction models, and/or a combination of any of these sources. Uncertainties in parameterization-based flux estimates are large, and when they are integrated over the ocean basins, they cause a large imbalance in the global-ocean budgets. Despite the significant progress that has been made in quantifying surface fluxes in the past 30 years, achieving a global closure of ocean-surface energy and water budgets remains a challenge for flux products constructed from all data sources.
In a recent review article, I provided a personal perspective on three questions: First, to what extent can time-series measurements from air-sea buoys be used as benchmarks for accuracy and reliability in the context of the budget closures? Second, what is the dominant source of uncertainties for surface flux products, the flux-related variables or the bulk flux algorithms (Figure 1)? And third, given the coupling between the energy and water cycles, precipitation and surface radiation can act as twin budget constraints-are the community-standard precipitation and surface radiation products pairwise compatible?
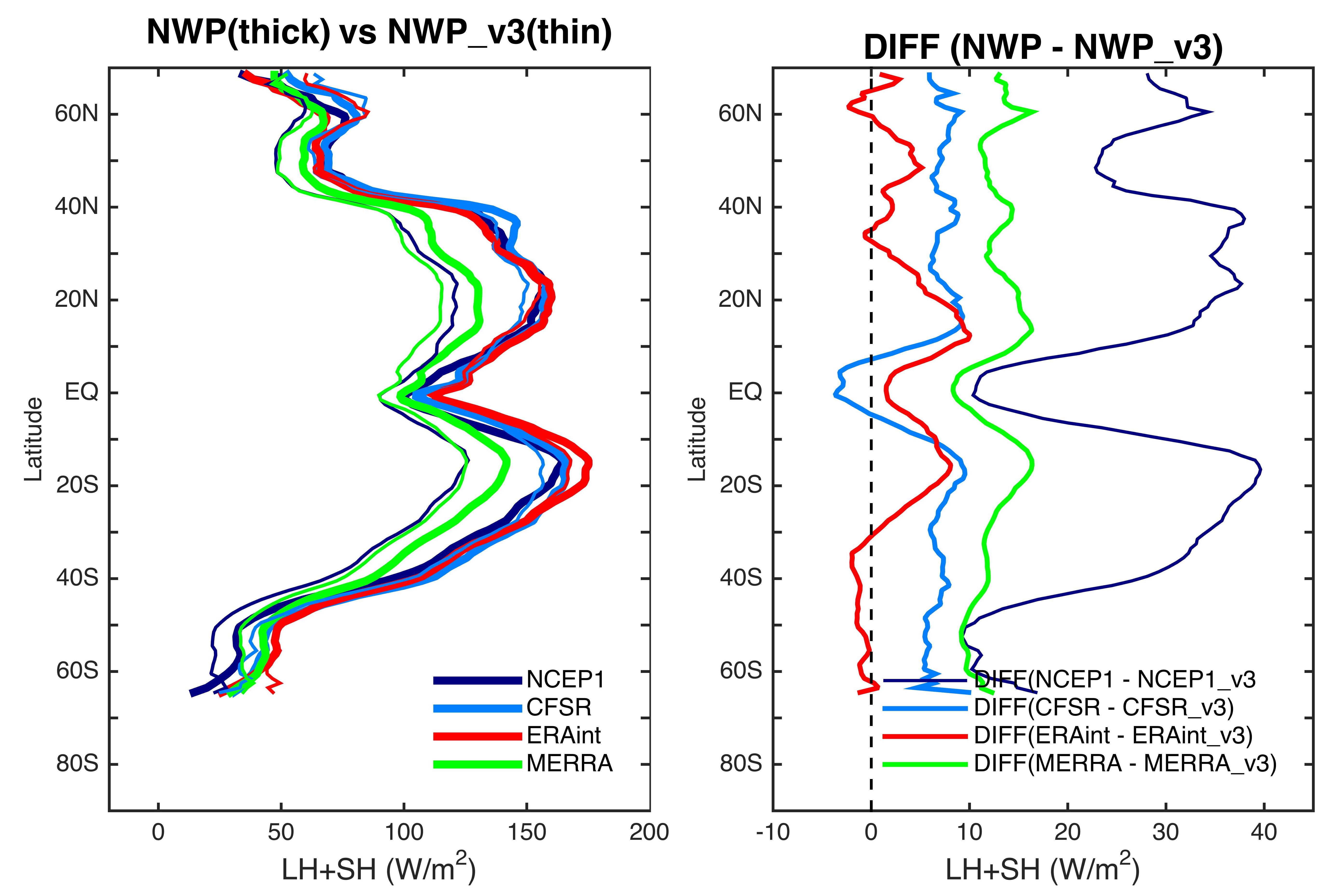